
실크 섬유의 주성분 분석 기반 물성 해석
© The Korean Society for Noise and Vibration Engineering
Abstract
Silk has excellent mechanical properties such as seven times the toughness of Kevlar 49 fiber; hence, it is considered as a candidate for the next generation fiber material. Although it is known that the crystalline region contributes largely to its mechanical properties, spider silk and silkworm silk use different amino acid sequences for the crystalline region. To determine the reason for the two types of silk to use different sequences and their effects, we compared two models representing respective silk types using principal component analysis and normal mode analysis.
Keywords:
Silk Fiber, Molecular Dynamic Simulation, Principal Component Analysis, Fiber Design키워드:
실크 섬유, 분자동역학 시뮬레이션, 주성분 분석, 섬유 설계1. 서 론
실크는 친환경, 생적합, 고성능 섬유로서 차세대 섬유재료의 후보 중 하나로 많은 연구가 진행되고 있는 단백질 섬유이다(1~4). 실크의 인성은 케블라 49 섬유의 7배 이상인 354 MJ/m3 이고, 인장강도(1.652 GPa) 는 고강도 철 (1.5 GPa) 보다도 높다. 이 실크는 스펀지, 하이드로젤, 필름, 매트, 마이크로니들 등 여러 형태로 재구성 될 수 있고(5), 따라서 실크는 넓은 범위의 어플리케이션에 기반 재료로 사용될 수 있다(6).
이런 실크를 대량 생산하기 위해 인공 실크 생산에 대한 연구가 많이 진행되었고, 그 결과로서 박테리아 같은 다른 생물에서 실크의 기반이 되는 단백질 재료를 생성하고, 방직기계를 통해서 대량으로 실크를 생산하는 연구 성과 등이 발표되었다(7,8).
그러나, 많은 인공 실크 생산에 대한 연구에도 불구하고, 인공 실크는 자연 실크의 물성에 미치지 못하였다. 따라서 자연 실크의 물성에 뒤처지지 않는 인공 실크의 생산을 위해서, 자연 실크의 높은 물성의 원리에 대한 분석이 필요하다.
이 방면으로 많은 연구가 진행되었고, 실크는 결정질 및 비결정질 영역으로 이루어져 있어서, 결정질 영역이 높은 강도로서 실크의 높은 물성에 지대한 기여를 한다는 것이 알려졌다(9).
결정질 영역의 높은 강도는 최적의 수소결합에 서 오는데, 이를 포함한 실크의 대부분의 특성은 실크의 아미노산 서열에 의해 결정된다. 실크의 아미노산 서열은 대부분 일정 구간이 반복되는 모티프 라고 불리는 아미노산 서열로 이루어져 있고, 이 모 티프들에 대해 연구하는 것을 통해 실크에 대한 효율적인 이해가 가능하다.
이 모티프들을 이해하기 위해 많은 연구가 진행되었으나, 아직도 많은 연구의 여지가 남아 있는 상태이다(10).
실크의 아미노산 서열 모티프 중 흥미로운 부분 중 하나는 거미 실크의 결정질 영역의 아미노산 서열 모티프와 누에 실크의 결정질 영역의 아미노산 서열 모티프이다. 거미 실크의 경우 결정질 영역의 대부분이 알라닌으로 이루어져 있는 대해 반해, 누에 실크의 경우 결정질 영역은 알라닌과 글라이신이 약 50 % : 50 %의 비율로 혼합되어 있다. 같은 실크 섬유지만 최적의 서열을 공유하는 것이 아니라 어째서 이런 차이가 존재하는지, 그 목적과 의미를 분석하고자 이 연구를 진행하였다.
이 연구에서는 이 모티프들 중 하나인 실크의 결정질 영역의 모티프에 대해서 분석하였다. 실크의 결정질 영역은 대체로 알라닌에 가끔씩 글라이신이 혼합된 구조인데, 가장 최적인 한 종류의 아미노산 이 아닌 두 종류의 아미노산이 혼합된 이유를 분자동역학 시뮬레이션을 통해 분석하였다. 주성분 분석을 통하여 글라이신과 알라닌이 혼합된 자연 서열, 그리고 알라닌만 있는 서열에 대해 진동 모드별 물성 해석을 진행하였다(Fig. 1).
2. 재료 및 방법
실크 모델은 글라이신과 알라닌이 혼합된 GA(Glycine-Alanine) 모델, 알라닌만 사용된 AA(Alanine-Alanine) 모델 2개 모델이 사용되었다. GA 모델은 PDB ID 2silk를 기반으로 적절한 크기가 되도록 복제시키는 것으로 만들어졌으며, AA 모델은 GA 모델을 UCSF Chimera(11)를 통하여 변이시키는 것을 통해 만들어졌다. 모든 시뮬레이션은 Gromacs 2019.3 시뮬레이션 패키지로 진행되었으며(12), AMBER99sb-ildn 역장과(13) TIP3P 물 분자 모델이 사용되었다. 모든 시스템은 NVT 조건에서 300 K, PBC(periodic boundary condition) 조건이 적용되었다. Time step은 0.002 ps로 진행되었고, 시뮬레이션 시간은 모델별로 10 ns 동안 진행되었다. 단거리 전기력 상호작용과 반데르바르스 상호작용은 cut-off 거리를 1.2 옴스트롱으로 진행하였고, 장거리 전기력 상호작용은 PME(particle mesh ewald) 방식으로 진행하였다(14). 시뮬레이션의 이미지화는 VMD 1.9.3 패키지를 통해서 이루어졌다(15).
각 진동 모드와 해당되는 물성은 시뮬레이션 결과에 대해서 주성분 분석 기법(16)을 적용하여 얻어졌다.
3. 결 과
주성분 분석을 통하여 GA 모델과 AA 모델의 진동모드를 분석하였고(Fig. 2), 그에 따른 진동 모드 별 물성의 해석을 진행하였다(Fig. 3).
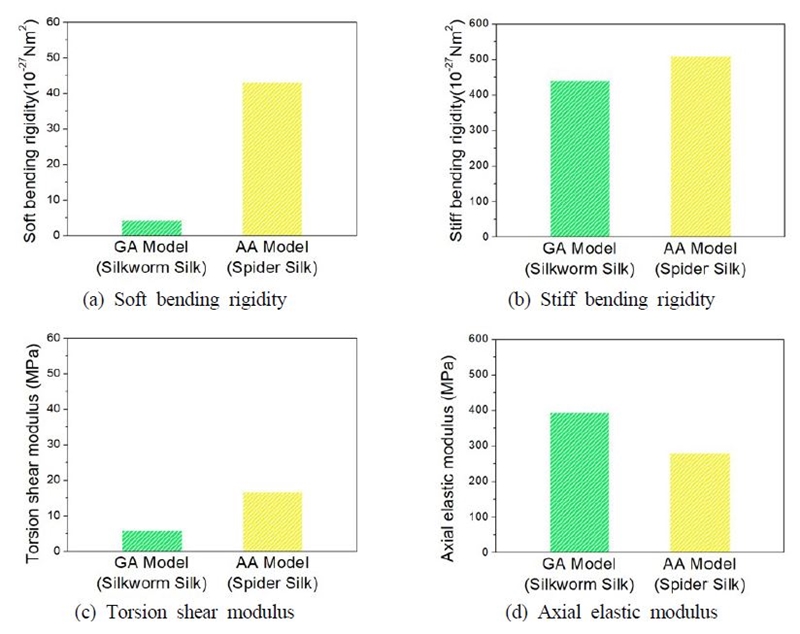
Mechanical properties from mode shapes of silkworm silk and spider silk obtained via principal component analysis
진동모드 해석 결과, soft bending 및 torsion에서 GA 모델이 AA 모델에 비해 더 극단적인 진동모드를 보여주는 것이 확인되었고. 이는 AA 모델이 해당 모드에 대하여 더 저항이 크다는 것을 암시하며, 이는 물성 해석 결과에서 뒷받침되었다.
물성 해석 결과, 누에 실크에 해당하는 GA 모델이 축방향 모드를 제외한 모든 모드에서 물성이 거미 실크에 해당하는 AA 모델 보다 낮게 분석되었다. 이는 진동모드 형상의 차이에서도 축방향 모드를 제외한 모드에서는 AA 모델이 GA 모델보다 최대 9.5배까지 강성이 높았으나, 축방향 모드에서는 GA 모델이 AA 모델보다 강성이 1.4배 높게 분석되었다.
이는 즉 AA 서열은 GA 서열에 비해 축방향 저항을 희생하여 다양한 모드에 대한 저항을 종합적으로 강하게 했다고 볼 수 있다.
이에 대한 해석은 거미 실크가 사용되는 목적과 누에 실크가 사용되는 목적의 차이에서 찾아볼 수 있다(Fig. 4). 거미 실크의 경우 거미 실크로 이루어진 그물에 주로 가해지는 하중은 그물에 대해 수직으로 가해지는 하중이다. 따라서 섬유의 입장에선 축방향 하중 뿐만 아니라 굽힘 강성 또한 강할 필요가 있다. 하지만 그에 비해 누에 실크의 경우 누에 고치의 형태로서 하중을 견디기 때문에 실크 섬유 사이의 연결 유지에 가장 연관성이 큰 축방향 저항을 가장 중시하는 것이 유리하다.
4. 결 론
이 연구에서는 실크 섬유의 종류별 아미노산 서열을 비교하여 그 특성의 차이 및 그에 따른 용도의 차이를 분석하였다.
전산해석 결과 거미 실크와 누에 실크는 상하적인 관계가 아니라 각 진동모드에 대하여 유리한 진동모드와 불리한 진동모드가 있다는 것을 밝혀내었다. 누에 실크는 축방향 강성이 강한데에 비해 거미 실크는 그 외 모든 강성이 강하였고, 이는 거미 실크와 누에 실크의 하중 환경과 일치하였다.
이 연구를 통해 실크의 종류별 아미노산 서열에 따라 진동 모드 별로 강점과 약점이 있다는 것과, 거미 실크와 누에 실크가 어떤 모드에 강한지를 알아 내었다. 이를 통해 실크 서열에 대한 더 깊은 이해를 얻었다.
Acknowledgments
2021년도 정부의 재원으로 한국연구재단의 지원을 받아 수행된 연구임(NRF-2019R1A2C1086103).
References
-
Pan, L., Wang, F., Cheng, Y., Leow, W. R., Zhang, Y.-W., Wang, M., Cai, P. Ji, B., Li, D. and Chen, X., 2020, A Supertough Electro-tendon Based on Spider Silk Composites, Nature Communications, Vol. 11, No. 1, pp. 1~9.
[https://doi.org/10.1038/s41467-020-14988-5]
-
Karan, S. K., Maiti, S., Kwon, O., Paria, S., Maitra, A., Si, S. K., Kim, Y., Kim, J. K. and Khatua, B. B., 2018, Nature Driven Spider Silk as High Energy Conversion Efficient Bio-piezoelectric Nanogenerator, Nano Energy, Vol. 49, pp. 655~666.
[https://doi.org/10.1016/j.nanoen.2018.05.014]
-
Widhe, M., Johansson, J., Hedhammar, M. and Rising, A., 2012, Current Progress and Limitations of Spider Silk for Biomedical Applications, Biopolymers, Vol. 97, No. 6, pp. 468~478.
[https://doi.org/10.1002/bip.21715]
-
Franco, A. R., Fernandes, E. M., Rodrigues, M. T., Rodrigues, F. J., Gomes, M. E., Leonor, I. B., Kaplan, D. L. and Reis, R. L., 2019, Antimicrobial Coating of Spider Silk to Prevent Bacterial Attachment on Silk Surgical Sutures, Acta Biomaterialia, Vol. 99, pp. 236~246.
[https://doi.org/10.1016/j.actbio.2019.09.004]
-
Zhou, L., Fu, P., Cai, X., Zhou, S. and Yuan, Y. 2016, Naturally Derived Carbon Nanofibers as Sustainable Electrocatalysts for Microbial Energy Harvesting: A New Application of Spider Silk, Applied Catalysis B: Environmental, Vol. 188, pp. 31~38.
[https://doi.org/10.1016/j.apcatb.2016.01.063]
-
Kiseleva, A. P., Krivoshapkin, P. V. and Krivoshapkina, E. F., 2020, Recent Advances in Development of Functional Spider Silk-based Hybrid materials, Frontiers in Chemistry, Vol. 8, p. 554.
[https://doi.org/10.3389/fchem.2020.00554]
-
Scheller, J., Gührs, K. H., Grosse, F. and Conrad, U., 2001, Production of Spider Silk Proteins in Tobacco and Potato, Nature Biotechnology, Vol. 19, No. 6, p. 573~577.
[https://doi.org/10.1038/89335]
-
Fahnestock, S. R. and Irwin, S. L., 1997, Synthetic Spider Dragline Silk Proteins and Their Production in Escherichia Coli, Applied Microbiology and Biotechnology, Vol. 47, No. 1, pp. 23~32.
[https://doi.org/10.1007/s002530050883]
-
Ene, R., Papadopoulos, P. and Kremer, F., 2009, Combined Structural Model of Spider Dragline Silk, Soft Matter, Vol. 5, No. 22, pp. 4568~4574.
[https://doi.org/10.1039/b911159j]
-
Malay, A. D., Arakawa, K. and Numata, K., 2017, Analysis of Repetitive Amino Acid Motifs Reveals the Essential Features of Spider Dragline Silk Proteins, PloS One, Vol. 12, No. 8, e0183397.
[https://doi.org/10.1371/journal.pone.0183397]
-
Pettersen, E. F., Goddard, T. D., Huang, C. C., Couch, G. S., Greenblatt, D. M., Meng, E. C. and Ferrin, T. E., 2004, UCSF Chimera—A Visualization System for Exploratory Research and Analysis, Journal of Computational Chemistry, Vol. 25, No. 13, pp. 1605~1612.
[https://doi.org/10.1002/jcc.20084]
-
Abraham, M. J., Murtola, T., Schulz, R., Páll, S., Smith, J. C., Hess, B., & Lindahl, E., 2015, GROMACS: High Performance Molecular Simulations through Multi-level Parallelism from Laptops to Supercomputers, SoftwareX, Vol. 1, pp. 19~25.
[https://doi.org/10.1016/j.softx.2015.06.001]
-
Lindorff‐Larsen, K., Piana, S., Palmo, K., Maragakis, P., Klepeis, J. L., Dror, R. O. and Shaw, D. E., 2010, Improved Side-Chain Torsion Potentials for the Amber ff99SB Protein Force Field, Proteins: Structure, Function, and Bioinformatics, Vol. 78, No.8, pp. 1950~1958.
[https://doi.org/10.1002/prot.22711]
-
Darden, T., York, D. and Pedersen, L., 1993, Particle Mesh Ewald: An N⋅log (N) Method for Ewald Sums in Large Systems, The Journal of Chemical Physics, Vol. 98, No. 12, pp. 10089~10092.
[https://doi.org/10.1063/1.464397]
-
Humphrey, W., Dalke, A. and Schulten, K. 1996, VMD: Visual Molecular Dynamics, Journal of Molecular Graphics, Vol. 14, No. 1, pp. 33~38.
[https://doi.org/10.1016/0263-7855(96)00018-5]
-
Yoon, G., Lee, M., Kim, J. I., Na, S. and Eom, K., 2014, Role of Sequence and Structural Polymorphism on the Mechanical Properties of Amyloid fibrils, PLoS One, Vol. 9, No. 2, e88502.
[https://doi.org/10.1371/journal.pone.0088502]
Yoonjung Kim is a graduate student in the Department of Engineering Science and Mechanics at Korea University.
Sungsoo Na is a professor in the Department of Engineering Science and Mechanics at Korea University. He received B.S. and M.S. in Engineering Science and Mechanics at Korea University and received Ph.D. in Engineering Science and Mechanics at Virginia Polytechnic Institute & State University.